Stephan Haas
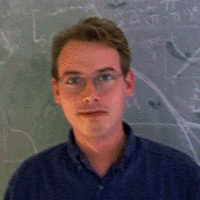
Email: | shaas@usc.edu |
Office Location: | SSC 211A |
Office Phone: | (213) 740-4528 |
Website: | Stephan Haas |
Ph.D. Programs: | Physics & Astronomy |
Our group is interested in topics related to quantum magnetism, superconductivity, and nanotechnology. We investigate microscopic models of interacting electronic systems, and use numerical techniques, such as Quantum Monte Carlo and Exact Diagonalization, to find their phase diagrams, ground state properties, and excitation spectra. Recently, we have applied the Stochastic Series Expansion Method to study field induced phase transitions in quantum spin liquids, developed optimization algorithms to construct nano-scale opto-electronic devices, and applied BCS theory to investigate the consequences of unconventional superconductivity in strongly correlated materials. Our work is motivated by and connected to recent experiments in the fields of quantum antiferromagnetism, high-temperature and heavy-fermion superconductivity, and nano-photonics.
More recently, we have also made several contributions to biophysics. In particular, we study how integration of back action into coupled rate equations describing dynamical biophysical processes can lead the identification of optimized structural features. This approach is used to analyze neural receptor binding and function. In functional receptor studies, the influence of ligand binding to the receptor on free ligand concentration in the synaptic cleft is rarely considered, especially when the number of ligand molecules vastly exceeds the number of receptors. We evaluate the role of ligand binding/unbinding to the receptor on ligand concentration and the resulting change in receptor dynamics using the example of glutamate interaction with the AMPA receptor subtype of glutamate receptors. We have found a significant difference for AMPA receptor-mediated current between the free diffusion case, where binding/unbinding is neglected, and the case when glutamate binding to AMPA receptors is taken into account for evaluating free ligand concentration. Furthermore, taking into account receptor binding/unbinding reveals new properties of the receptor/neurotransmitter system, and in particular, indicates the existence of an optimum receptor density profile with an optimal radius where the total charge and peak current are maximal, a property that cannot be captured by the free diffusion case. This may provide an explanation for the disposition of AMPA receptors and the synaptic geometry based on the optimization of the receptor-mediated current.
We have also devised a new simulation technique, based on a combination of molecular dynamics and Jarzynski’s equality to study ion transport through cellular membranes. The ability of biological ion channels to conduct selected ions across cell membranes is critical for the survival of both animal and bacterial cells. Numerous investigations of ion selectivity have been conducted over more than 50 years, yet the mechanisms whereby the channels select certain ions and reject others are not well understood. Here we study a new application of Jarzynski’s Equality to investigate the mechanism of ion selectivity using non-equilibrium molecular dynamics simulations of Na+ and K+ ions moving through the KcsA channel. Our simulations show that the selectivity filter of KcsA adapts and responds to the presence of the ions with structural rearrangements that are different for Na+ and K+. These structural rearrangements facilitate entry of K+ ions into the selectivity filter and permeation through the channel, and rejection of Na+ ions. A mechanistic model of ion selectivity by this channel based on the results of the simulations relates the structural rearrangement of the selectivity filter to the differential dehydration of ions and multiple-ion occupancy and describes a mechanism to efficiently select and conduct K+. Estimates of the K+/Na+ selectivity ratio and steady state ion conductance for KcsA from the simulations are in good quantitative agreement with experimental measurements. This model also accurately describes experimental observations of channel block by cytoplasmic Na+ ions, the “punch through” relief of channel block by cytoplasmic positive voltages, and is consistent with the knock-on mechanism of ion permeation.