Naggar lab: Biological Electron Transport
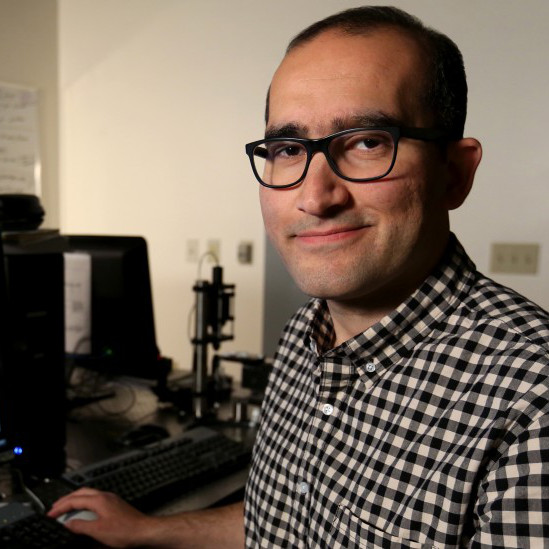
Email: | mnaggar@usc.edu |
Office Location: | SSC 215C |
Office Phone: | (213) 740 2394 |
Lab Location: | SSC 107 and SSC 112 |
Lab Phone: | (213) 740 6838 |
Ph.D. Programs: | Physics |
Long-Range Charge Transport in Microbial Systems: Biofilms and Bacterial Nanowires
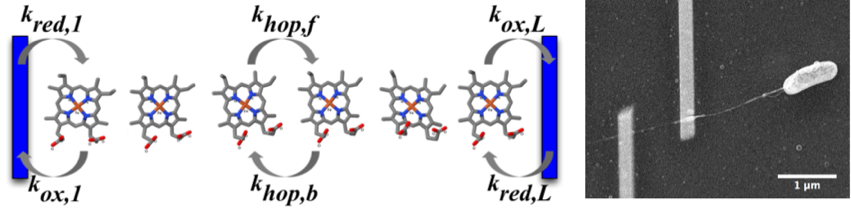
The recent discovery that metabolically diverse bacteria produce electrically conductive appendages is rapidly reshaping our understanding of extracellular electron transfer in microbial communities. We’ve initiated an interdisciplinary research program to investigate the molecular building blocks and the basic physics of conduction in biofilms and bacterial nanowires. Our improved understanding of this topic has immense implications for physiology, ecology, bioenergy production, bioremediation, pathogenic biofilms, and signal transduction across the biological-inorganic interface. To describe long-range charge transport in these microbial systems, we are developing theoretical models and experimental systems based on incoherent hopping between redox sites in molecular assemblies, combined with an interfacial treatment of electrochemical interactions with measurement electrodes. With this basic physical picture, we recently calculated the micrometer-scale current-voltage (I-V) characteristics and found the results to be in good agreement with our I-V measurements across and along individual nanowires produced by the bacterium Shewanella oneidensis MR-1. Based on our analysis, we propose that multistep hopping in redox chains constitutes a viable strategy for extracellular charge transfer in microbial biofilms, with significant implications for energy harvesting technologies.
The NASA Astrobiology Institute (NAI) Team at USC: Life Underground
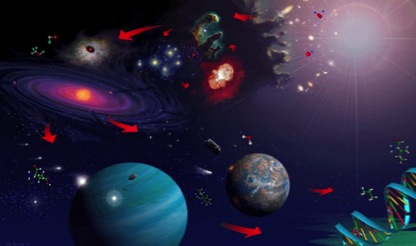
On Earth, microorganisms inhabit all physical space that provides the minimum requirements for life, including the availability of water, carbon, nutrients, and light or chemical energy. While these are generally abundant in surface or near-surface environments, we now also know that archaea and bacteria inhabit deeply buried rocks and sediments, and all evidence suggests that these subsurface ecosystems are spatially enormous and diverse. On other planets, at least in our solar system, putative extant or extinct life would most likely reside underground or in massive ice shells. The Life Underground NAI team, of which our laboratory is a part, is developing field, laboratory, and modeling approaches aimed at detecting and characterizing microbial life in the subsurface - the intraterrestrials. This study will inform the astrobiology community and guide future missions in the search for extraterrestrial life. We are taking full advantage of rare access to terrestrial deep subsurface sites, and are developing in situ life detection technologies and new cultivation techniques. As part of this effort, the El-Naggar laboratory is developing on-chip platforms that mimic the energetic conditions as well as inter-species and biotic-abiotic (e.g. microbe-mineral) interactions found in the subsurface. These new platforms will be used to cultivate new subsurface microbes and to study the mechanisms of biotic-abiotic energy transfer that these organisms have evolved to overcome the obstacles of low energy environments.
Biological Routes to Nanomaterial Synthesis
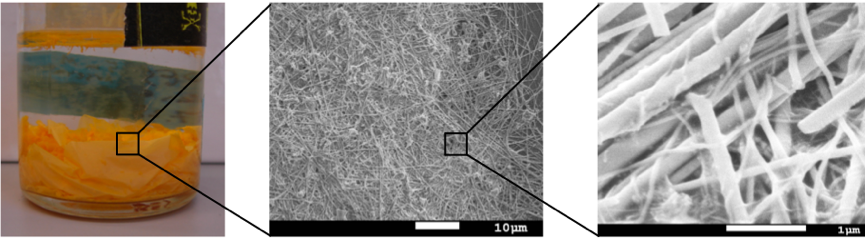
Taking advantage of the respiratory and detoxification activities of microbes offers a potentially transformative approach to the biogenic synthesis of nanostructured inorganic materials for semiconductor, optical, optoelectronic, and optomechanical applications. In one recent demonstration, the bacterium Shewanella oneidensis MR-1 drives the synthesis of semiconductive arsenic sulfide nanofibers (pictured here). Our understanding of Shewanella's genome and metabolism, coupled with our experience in nanomaterial synthesis and characterization, enables us to explore the molecular basis of nanostructure formation, and the potential to synthesize other technologically important chalcogenide materials. The production of nanomaterials by biological means opens the possibility of cheaper manufacturing technologies in mild environmental conditions (temperature, pressure, and pH), while taking advantage of the exceptional control organisms exert over the composition, crystallography, and functional properties of biogenic materials
Bioenergy from Microbial Fuel Cells
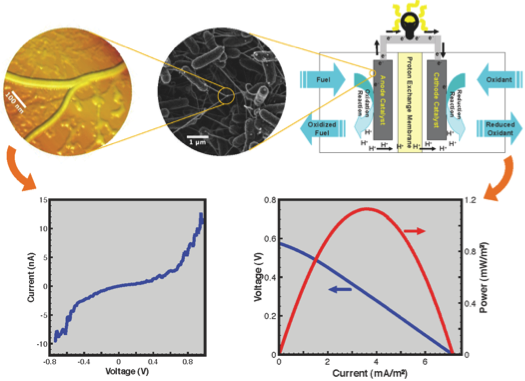
The ability of certain microorganisms to transfer electrons directly to solid surfaces enables the emerging technology of microbial fuel cells, where bacteria are used as catalysts to convert fuel to electricity. Understanding the electron transport physics at the biological-inorganic interface is critical to improving the power density of fuel cells that function directly by consuming biomass. Our objective is to understand how nanoscale microbial-surface interactions translate to the observable power output in these devices. To accomplish this goal, our approaches combine surface modification, scanning probe microscopy, nano-scale electrical characterization, and macro-scale electrochemical analysis. This work is part of a Multidisciplinary University Research Initiative (MURI) and is funded by the Air Force Office of Scientific Research.